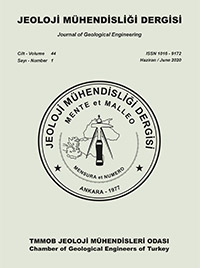
ABSTRACT:This study evaluated the potential rockfall hazards in the Kayseri-Soğanlı settlement using the energy angle method and 3-dimensional probabilistic rockfall modeling. The high-resolution digital surface model (DSM) was obtained with the help of unmanned aerial vehicles (UAV) and high-resolution orthophoto. 3-dimensional probabilistic rockfall simulations were conducted on the DSM using the CONEFALL software, and rockfall zones were determined according to the energy line angle method. The dimensions of previously fallen rock blocks were determined using fieldwork and orthophoto generated by UAV. As a result of 3-dimensional probabilistic analyses, the maximum kinetic energy values of 15,000 kJ and bounce height of 15 m were determined during the movement of 3-meter rock blocks along the slope. When the energy line angle method was considered, it was found that the previously fallen blocks were mostly concentrated in the 40ο energy line angle zone in the southern part of the slope.
On the other hand, although the rolling lines obtained from probabilistic 3- dimensional rockfall analyses for 3 metre block size reach up to the maximum 30ο energy line angle zone, these rolling lines end in the 40ο energy line angle zone, especially in the southern part of the slope. Therefore, the empirical method, 3-dimensional probabilistic method, and the positions of measured blocks in the field show general consistency.
ABSTRACT: Flooding is one of the natural disasters that occur with changes in meteorological conditions such as excessive precipitation and the rapid melting of snow. The major flooding formation factors are a regions topography, geological structure, climatic conditions, any deterioration in the natural structures of rivers, and uncontrolled urbanization. To reduce the risk of flooding, this study conducted river rehabilitation work within the water collection basin of the Yazılıkaya River, located within the borders of the Nallıhan District of Ankara Province. Furthermore, a mortared check dam was designed and implemented in an area of 78.8 hectares (Ha). The study of the Yazılıkaya River in-stream rehabilitation project included a two-dimensional flood model, which was carried out with the help of HEC-RAS software (Hydrologic Engineering Centers River Analysis System) using obtained data (stream maps, flow rates and art structure cross-sections) and the DSI Synthetic method with Q10 and Q100 flow rates. To analyze the effects of a mortared check dam on the Yazılıkaya River, the flow rate values of Q10 and Q100 in both heavy and sudden rains were used; it was observed that while the speed of the water was 2-3.2 m/sec before the construction of the dam, it decreased to 0.5 0.75 m/sec after construction. After the completion of the mortared check dam, it was calculated that there were water reductions of 2 and 8 cm respectively in the water depth analysis in the settlements made according to the Q10 and Q100 flow rates. Generally, this study investigated the effect of upper basin flood control structures (mortared check dam) and the regulation of precipitation water flow regimes (water energy, speed, depth, etc.), on flood hazard in populated settlements and on the highway.
ABSTRACT: On February 6, 2023, two distinct earthquakes occurred approximately 9 hours apart in the districts of Pazarcık and Elbistan in Kahramanmaraş. The first earthquake had a magnitude of Mw 7.7; and was followed by a second earthquake with a magnitude of Mw 7.6. These independent seismic events were felt over a wide area and caused significant damage in the epicentral region and surrounding provinces. Numerous buildings collapsed and according to official records, the earthquakes resulted in the loss of more than 50,000 lives. The most affected cities include Adana, Adıyaman, Diyarbakır, Gaziantep, Hatay, Kahramanmaraş, Kilis, Malatya, Osmaniye, and Şanlıurfa; most of the destruction and significant loss of life occurred here. This study has compiled the building stock in these provinces. The examined building stock has been gathered on a district basis, considering each buildings structural system, building use, number of floors, and seismic code time periods. Buildings that collapsed, suffered severe damage, or were condemned (to be demolished) after the earthquakes are no longer included in the compiled building stock.However, the recorded ground motion acceleration data obtained during the earthquakes from various strong-motion stations; and the damage level information regarding the buildings in the stock, constitute an important dataset for earthquake studies. In this context, conducting earthquake risk assessments for the pre-earthquake building stock, esting and calibrating fragility curves appropriate for the building types within the building stock, is possible. It is believed that the information presented in this study will make a significant contribution to the realistic calculation of earthquake vulnerability and thus assist in taking measures to mitigate earthquake risks. Furthermore, utilizing the compiled building stock, it is also possible to perform loss calculations for similar building stocks exposed to seismic hazards in different regions.
ABSTRACT: Earthquake ground motion on a site depends on the fault rupture mechanism, the structure of the crust, and the properties of the near-surface soils and soft rocks. It is known that near-surface soils and soft sedimentary rocks can cause significant structural damage even at great distances from the earthquake source. This phenomenon was observed in recent earthquakes in our country. The 6.9 magnitude earthquake that occurred off the coast of Samos Island in the Aegean Sea on October 30, 2020, caused extensive damage in the İzmir Bayraklı district, which is approximately 70 km from the epicenter. On February 6, 2023, the 7.7 moment magnitude Kahramanmaraş Pazarcık earthquake caused great loss of life and destruction in large areas. The response spectra of the ground motions created by the Kahramanmaraş Pazarcık earthquake in the Antakya region of Hatay, were far above the design response spectra defined in the Turkish Building Earthquake Code. In this study, the site effects affecting the earthquake ground motions and the near-fault effects are summarized. Some of the ground motion records recorded during the 7.7 magnitude Kahramanmaraş Pazarcık earthquake in the Hatay region were examined and evaluated in terms of possible basin effects and near field effects.
ABSTRACT:Materials produced in rock quarries have many uses, such as concrete and cement raw material, asphalt and embankment aggregates and railway ballast. Aggregate production activities, which are mostly carried out in urban areas, are a risky activity due to geological, environmental, social, legal and economic uncertainties. Projects based on technical and scientific data have reduced uncertainty and risks; furthermore, projects developed with transparent and consistent data, will ensure that all internal and external stakeholders have accurate information about project risks.
The quality expectations of rocks change according to their planned use. Many quarries exhibit parametric differences within very short distances. For example rock composition and texture, organic material and shell content, structural characteristics, weathering types and their products, and the presence of dangerous substances can all vary. For this reason, pre-operation geology and engineering geology studies should be carried out in the quarries.Determining the composition and quality changes of the rocks in the quarries and the geological, hydrogeological, geotechnical and environmental risks will play an important role in the quarry and facility planning. The aim of this study is to emphasize the importance of detailed geology and engineering geology surveys in reducing uncertainty and risks in quarry areas.